TM-30 the new measure of color - the better Color Rendering Index (CRI)
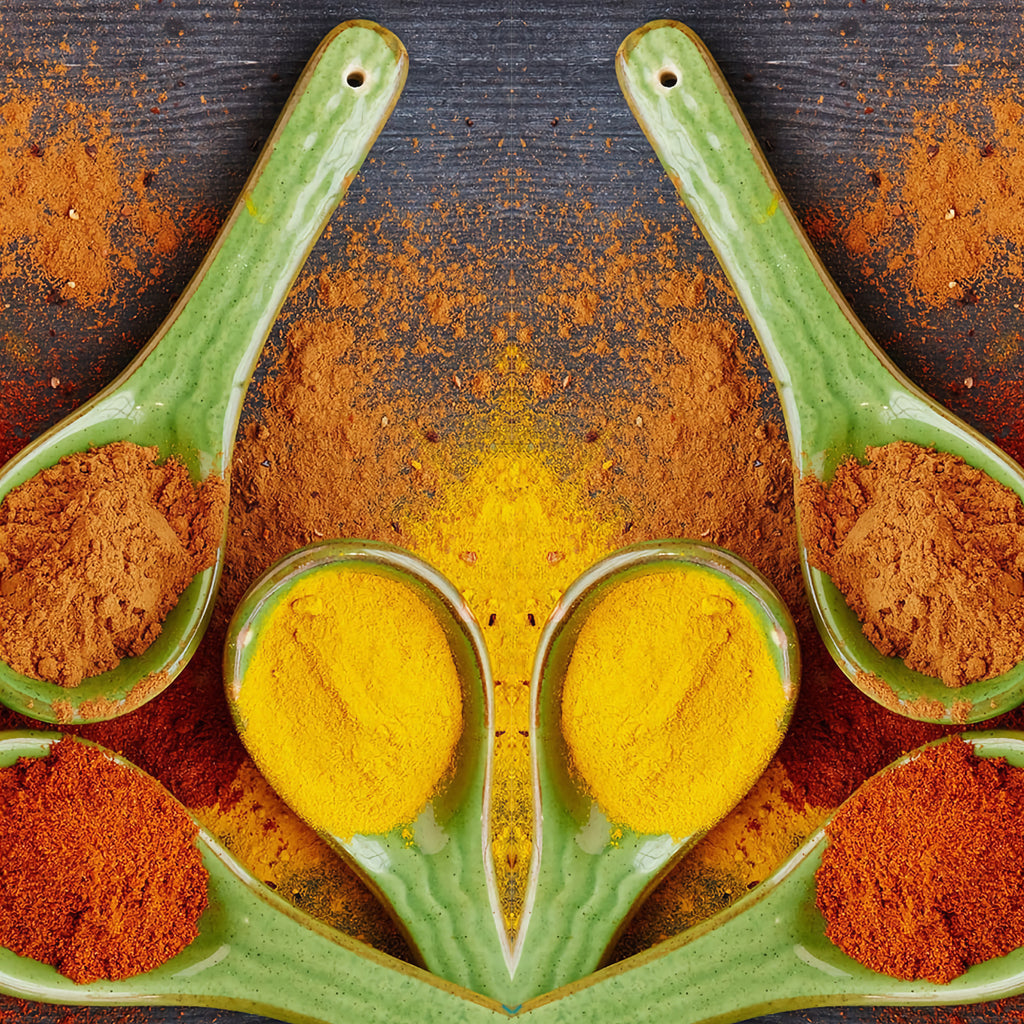
The new standard for the colour rendering index
Together with scientists from SORAA Inc. and the Illumination Engineering Society (IES), a new and improved method for measuring the colour rendering quality of light sources has been developed.
TM-30 is a highly precise and scientifically sound method and surpasses the previous method for determining the so-called CRI
Physics
TM-30 takes into account the latest scientific findings in optical physics describing light and light colours.
The result is much more meaningful than the CRI value alone.
Precision
TM-30 provides far more precise information, such as an index for the vividness (Rf) of colour rendering.
This value replaces the previous CRI index. The new index enables a clear prediction of how true-to-life colours appear.
More insight
TM-30 also offers more insight into the so-called gamut index (Rg).
This index allows the graphical representation of colour saturation and the precision achieved in the representation of each individual colour.
In a previous BLOG post, I described the CRI and its test colours.
Here we want to go into this in more depth and look at the factors that can influence the colours of an object.
In general, a mixture of hue shift and saturation shift (more or less saturation) can occur. As we have seen, the CRI is only interested in the absolute magnitude of this shift and therefore gives no information about the type of shift - although this information is relevant for our perception. Today I would like to explain how the TM-30 provides this additional information.
Let's first look at how we can visualise colour shifts in a colour space. A colour space is simply a space in which all the colours we can see are categorised.
There is some maths involved, but the interpretation is more intuitive. The figure below shows a sketch of a colour space; different colours are characterised by their hue and saturation. The third dimension is not shown for the sake of simplicity: it corresponds to brightness, i.e. whether a colour is dark or light. The natural colour of an object illuminated by a light source corresponds to a point in this diagram; a light source (e.g. an LED) can cause a colour shift, making the object appear at a different point. Figure 1 shows the example of an LED light source that makes red objects more saturated (positive saturation shift) and orange objects appear yellowish (hue shift) - all compared to natural colours. As we can see, by drawing arrows in the colour space, we can see how the LED source distorts the colours.
Figure 1, left: Sketch of a colour space. The saturation increases from the centre to the edge of the diagram. In this example, the colour coordinates of two objects (a tomato and an orange) are plotted under natural light and under LED light. The LED light increases the saturation of the tomato (so that it looks riper) and shifts the colour of the orange towards yellow (so that it looks more like a lemon). The corresponding appearance is shown on the right.
This is exactly how the TM-30 method works:
It looks at ninety-nine real objects with a variety of colours - these have been carefully selected according to their reflective properties, but that's a story for another time! At this point, the colour palette of the TM30 method is only shown... in comparison, the 14 test colours of the CRI are manageable 😉
The colour shift caused by the relevant light source is calculated for each object. The objects are then grouped into colour tone ranges and an average shift is calculated for each range. In this way we obtain the diagram in Figure 2, which is called a colour vector diagram and is one of the results of TM-30. This graph shows how the different colours are distorted on average. The visual interpretation is intuitive: arrows pointing outwards mean more saturated colours, arrows pointing inwards mean duller colours, arrows pointing sideways mean a hue shift, and no arrow means that the colour is not distorted!
Figure 2: Colour vector graphics from TM-30. The arrows show how colours are distorted from their natural colours (which lie on the white circle) by different light sources. Low quality LEDs desaturate various colours, especially red and other warm tones, and make them appear dull: they are characterised by a low Rf and a low Rg. SORAA Vivid reproduces natural colours and causes hardly any distortion: It is characterised by a high Rf value. SORAA Enhance selectively increases the saturation of warm tones: It is characterised by a medium Rf value and a high Rg value.
However, a few things are generally true.
- We don't like desaturated colours, especially warm colours (red, pink, orange)
- We don't like hue shifts because they make the colours look unfamiliar
- In some cases we want colours to look natural - in this case we don't want any colour shifts.
- In other cases, we like overly saturated colours (again, this is especially true for warm colours)
This leads us to the next step in TM-30, which is to reduce this graph to just two numbers for simplicity. The first number is the colour fidelity index Rf. Colour fidelity means "no colour distortion". Rf simply measures the average length of the arrows in the colour vector graphic. If all arrows have a length of zero, the colours are "natural" and Rf assumes a maximum value of 100. When colour shifts occur, the arrows become longer and Rf decreases (to a minimum value of zero). This is similar to the CRI Ra - but the more precise and modern science in the TM-30 makes the prediction of Rf more accurate.
The second number is the gamut index Rg, which indicates whether a light source on average oversaturates or undersaturates colours. Rg is calculated as the area of the shape connecting the tips of the arrows. A value of 100 means that the area of the shape is the same as under natural light - in other words, no average change in saturation. A value above 100 means that the saturation tends to increase, and vice versa for Rg below 100.
It is important to understand that Rf and Rg are closely related. If Rf is equal to 100, there is no colour shift; in this case, Rg must also be equal to 100. On the other hand, if Rf decreases, there will be a colour shift and Rg can assume a value above or below 100. Roughly speaking, we can exchange a point Rf for a point Rg. So if we want a "colour enhancing" source with Rg=120, Rf must be at or below 80. In other words, nothing comes for free: higher saturation must come at the expense of colour fidelity!
Therefore, light sources can be arranged on a diagram with Rf-Rg values as shown in Fig. 3.

Figure 3: Illustration of the trade-off between Rf and Rg. Sources can only be located in the non-grey region. Three zones are of particular interest: in green, the high-fidelity zone, where SORAA Vivid is located; in red, the high-gamut zone (colour gamut) of SORAA Enhance; in blue, the lowlands of products with poor colour quality.
In this illustration, I show several regions. The three regions of interest are:
- The low-fidelity/low-gamut zone that nobody really likes, but where many products can be found because it is easy to make such light sources more efficient in terms of lumens per watt.
- The high-fidelity zone Rf, which corresponds to high-CRI products such as SORAA Vivid and has a clear appeal, but is associated with a slight reduction in efficiency (lumens/watt).
- The high-gamut zone Rg, which "intensifies" the colours, which can be desirable in some applications.
A word of warning on the latter:
Rg tells you that some colours will be boosted, but it doesn't tell you which ones! Ultimately, users of sources that boost saturation need to look at the details of the colour distortion graph to really know what they are getting in the end, or which colours and colour ranges are affected.
All of this is to help you understand one of the problems with the simple CRI value. We sometimes assume that a low CRI (or a low Rf) is bad per se. This is not always the case: sometimes a source with moderate Rf and high Rg can be pleasant.
But basically, most sources on the market today with a low CRI also have a low Rg value because they favour lumens over colour or colour fidelity. Or to put it another way, the completeness of the colour spectrum and a high CRI value for all these individual colours costs efficiency. The lumen/watt yield is therefore metrologically lower than with a low CRI or an LED that only displays a few colours with high rendition quality.
That was quite a lot of material on how to understand the TM-30 - but I hope I have shed some light on the subject.
For further reading, the official TM-30 document can be downloaded here. The scientific article describing the development of the TM-30 is freely available here, otherwise please feel free to contact us.
The complete range: SORAA Full Spectrum LED
Book a free consultation appointment now!